The lab of molecular-structural biology, headed by Associate Professor Tali Haran investigates the recognition mechanisms of DNA by proteins. Our research probes the mechanism of Protein-DNA interaction in cellular function.
On the DNA double helix are encoded several codes simultaneously: the genetic code, the code for packaging DNA within nucleosomes, and the code for interaction between defined DNA elements and regulatory proteins. All codes coexist on the same DNA region, and hence the codes must be degenerate, meaning that there should be more than one option to specify the encoded massage. The code for packaging into nucleosomes and for protein-DNA interactions reside in the structural properties of DNA base-pair combinations, and therefore the code is intrinsic to the conformational properties of particular DNA sequences.
Thus, the interests of the Lab are in deciphering the mechanism by which DNA structure contributes to the recognition of particular sites by regulatory proteins (termed indirect readout). For the last years with a focus on p53 – an important protein known as the “cell genome keeper because of its tumor suppressor activities. p53 is a transcription factor that binds to its DNA targets as a tetramer (Figure 1). The tetrameric p53 binds cooperatively to specific DNA response elements (REs) upstream of a gene , composed of two decameric half-sites of the general form RRRCWWGYYY (R=A,G;W=A,T;Y=C,T), separated by a 0-18 base-pair linker (Figure 2).
It was earlier shown by us (Kitayner et al 2006) that in p53/DNA complexes, the protein-DNA interface varied as a function of the DNA base sequence, and that there is a correlation between binding affinity of the complexes and the protein-DNA interface geometries. We then showed that changes in torsional (twist) flexibilities of p53 response elements are the most significant structural difference between p53 response elements (Beno et al 2011, Jordan et al. 2012, Menendez. et al. 2012) This opened the way to a multitude of questions, which are the focus of our current research (read more about projects conducted in the lab).
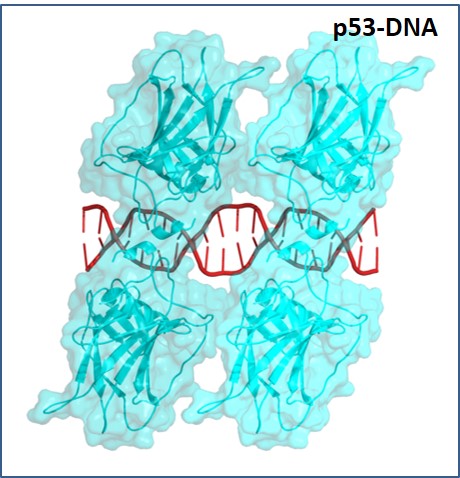
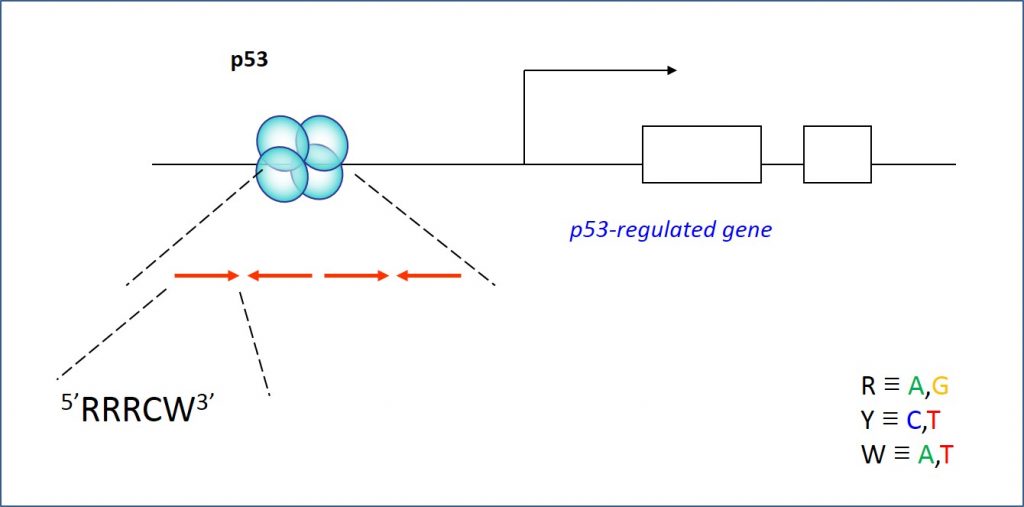
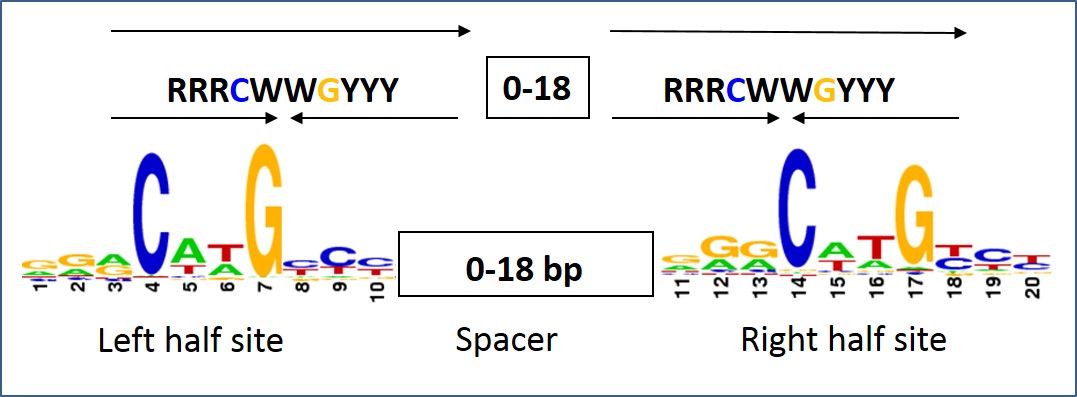
Using a combination of in vivo transactivation and in vitro physical analysis of the interactions, we described two sets of relationships (Jordan et al. 2012 Menendez et al. 2012) as presented in figure 3: at highly induced p53 levels, transactivation followed the binding affinity of the sequences to p53. However, at basal p53 levels transactivation is correlated with the torsional flexibility of target sequences (Figure 3). Torsional flexibility of the response elements (RE) can contribute to the kinetic stability of p53 dimers on the RE, because it can facilitates the reorientation of two p53 monomers within each dimer, optimizing their stability.
Thus, our findings establish that p53 transactivation is kinetically determined at low levels and thermodynamically driven at high levels. Moreover, we showed that the torsional flexibility of unmeasured sequences can be estimated using the dispersion of twist angle values from their average values, as determined from crystal structures of protein–DNA complexe (Beno et al. 2011, Jordan et al. 2012). (read more about the method used in lab).
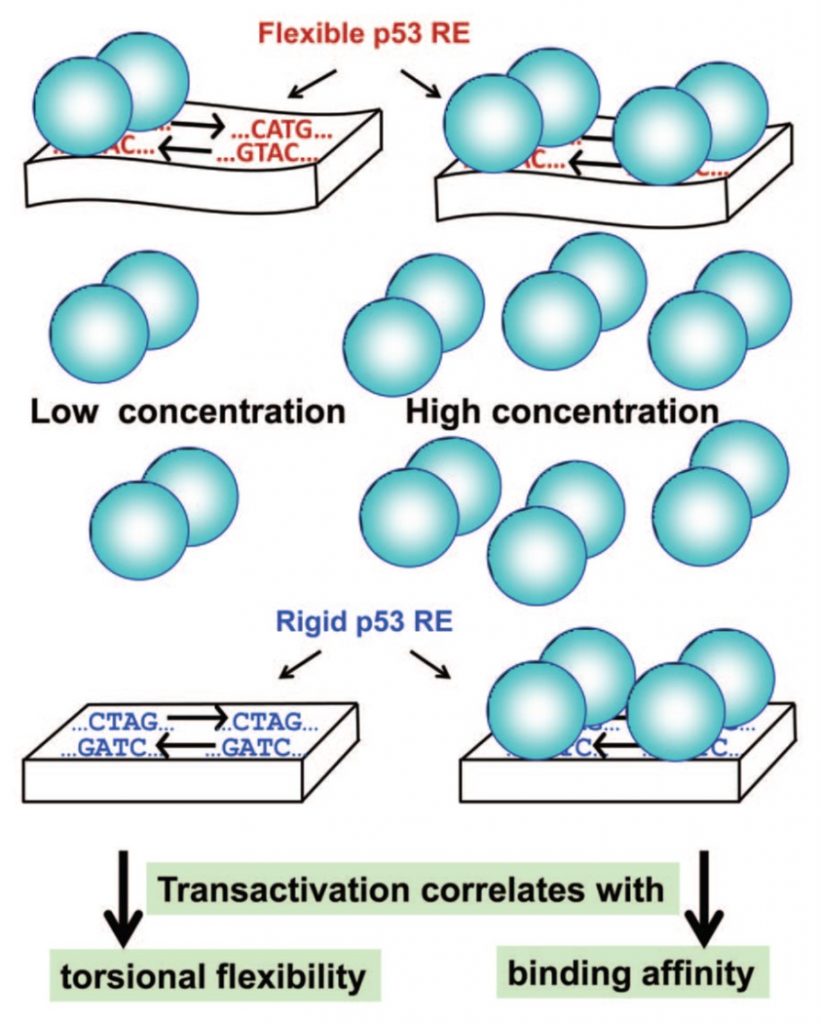